A History of Mathematical Astronomy - Part 2
From the desk of Fjordman on Fri, 2010-01-08 10:09
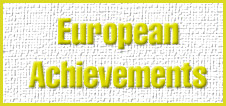
Ancient Greek planetary theory was brought into its final, very successful form with Ptolemy’s masterpiece in Alexandria in the second century AD. James Evans explains in his book The History and Practice of Ancient Astronomy:
“The original title was something like The 13 Books of the Mathematical Composition of Claudius Ptolemy. Later the work may simply have been known as Megale Syntaxis, the Great Composition. The superlative form of the Greek megale (great) is megiste. Arabic astronomers of the early Middle Ages joined to this the Arabic article al-, giving al-megiste, which was later corrupted by medieval Latin writers to Almagest….The Almagest is one of the greatest books in the whole history of the sciences – comparable in its significance and influence to Euclid’s Elements, Newton’s Principia, or Darwin’s Origin of Species.”
The mathematician al-Battani (ca. 850-929) made measurements of the stars and planets. The Persian astronomer Abul Wafa (940-998) was a capable mathematician who made good trigonometric tables. Ibn Yunus (950-1009), an Egyptian mathematician, made reliable observations of the Moon and described many lunar eclipses. A lunar crater has been named in his honor and another one in honor of Ibn al-Zarqali, Latinized as Arzachel, an eleventh-century Andalusian astronomer who was partly responsible for the so-called Toledan Tables. These were accurate for their time and were later translated into Latin and used in Europe.
The Mongols under the leadership of Hulegu Khan (ca. 1217-1265), a grandson of the feared and powerful Mongol conqueror Genghis Khan (ca. 1162-1227), sacked Baghdad in 1258. Hulegu believed that many of his military successes were due to the advice of astronomers who were also astrologers (astrology was important in Mongol culture) and was persuaded to found an observatory in Iran by the talented Persian mathematician Nasir al-Din al-Tusi (1201-1274). Muslim achievements in astronomy thus peaked after the Mongol conquests.
Astronomy in the Islamic world remained fundamentally Ptolemaic and Earth-centered, but Ptolemy did have critics regarding certain technical details. The planetary models developed by Maragha astronomers such as Ibn al-Shatir of Damascus (ca. 1304-1375) showed some originality. Some mathematical constructions similar to those of Ibn al-Shatir later turned up in the work of Nicolaus Copernicus, who may have learned of them while studying in Italy.
The Maragha observatory from 1259 was destroyed already in the early 1300s. According to author Toby E. Huff, “The fact that the Maragha observatory not only stopped functioning within fifty years but soon thereafter was completely obliterated suggests that there were very strong antipathies against it and its activities” because of their alleged association with astrology, which was considered a challenge to the omnipotence of Allah. The observatory as a scientific institution failed to take root in the Islamic world due to religious resistance. As historian Bernard Lewis states, in the Ottoman Empire the observatory in Constantinople/Istanbul created by Taqi al-Din (1526-1585) “was razed to the ground by a squad of Janissaries, by order of the sultan, on the recommendation of the Chief Mufti.”
Among major regions or civilizations, the two with the most similar medieval starting points were the Middle East and Europe. Greek geometry was virtually unknown in East and Southeast Asia. This constituted a major disadvantage for Chinese, Japanese and Korean scholars in optics and astronomy. The only regions where clear glass was extensively made were the Middle East and Europe. Clear glass was used by Europeans to create eyeglasses for the correction of eyesight and later for the creation of microscopes and telescopes, facilitating the birth of modern medicine and astronomy. The Maya in pre-Columbian Mesoamerica did not know how to make glass and could consequently not have made glass lenses for microscopes or telescopes. Muslims could have done so, but they didn’t. Likewise, medieval Europeans invented mechanical clocks while Muslims did not, despite a similar starting point.
The best Muslim scholars could be capable observational astronomers and a few made minor adjustments to Ptolemaic theory, but none of them ever made a huge conceptual breakthrough comparable to that provided by Copernicus when he put the Sun, not the Earth, at the center of our Solar System. Combined with the pre-telescopic work of Tycho Brahe and Johannes Kepler, Ptolemaic astronomy was in reality outdated in Europe even before Galileo had introduced telescopic astronomy by 1610. In contrast, Muslims resisted Copernican heliocentrism in some cases into the twentieth century. One of those who rejected it was the influential late nineteenth century pan-Islamic alleged reformist Jamal-al-Din al-Afghani.
As Toby E. Huff says in his fine work The Rise of Early Modern Science,
“Sayyid Ahmad Khan (1817-98) was at the forefront of intellectual reform in India, encouraging India to adopt Western educational standards. In his early career of the 1840s, he had defended the Ptolemaic view against Copernicanism, believing that this was incumbent upon the devout Muslim. As he studied the matter more, he realized the need to adopt heliocentric view and to reconcile its metaphysics with traditional interpretations of the Quran. Soon after he moved to adopt the heliocentric position, he ran into overwhelming opposition, especially Jamil al-Din al-Afghani's (d. 1897) attack of the early 1880s.”
The greatest astronomical advances in human history began in Europe following the Renaissance period. The translation of Greek and Latin Classical works, dictionaries and texts culminated in printed editions at the turn of the sixteenth century. Many of these were written, translated or edited by Byzantine émigré scholars such as Cardinal Johannes Bessarion (1403-72), a Byzantine Greek with excellent personal connections who promoted the study of the classics of Greek literature and philosophy in Western Europe after the Ottoman conquests.
The number of competent, practicing astronomers in late medieval European universities far exceeded the number who had been active at any stage during Greek Antiquity. One of them was the Austrian Georg Peurbach, who was born near the city of Linz on the river Danube. He travelled through Europe around 1450 and after that accepted a chair at the University of Vienna. Peurbach published observations as well as a textbook on trigonometric calculation.
The German astronomer Johannes Müller was born near Königsberg in Bavaria and Latinized his name to Regiomontanus. He was a talented mathematician who worked squarely in the Ptolemaic tradition. He was educated at the Universities of Leipzig and Vienna. In Italy he perfected his Greek and read widely in Bessarion’s library. The Epitome of the Almagest was completed around 1463 but printed in 1496. In 1464 Regiomontus published the first systematic European work on trigonometry as a subject divorced from astronomy. Between 1467 and 1471 he worked in Hungary for King Matthias Corvinus (1443-1490), a great patron of the arts and sciences and son of the Hungarian general John Hunyadi (ca. 1400-1456) who led the Christian European defenses against the aggressive Turks. In 1471 Regiomontanus relocated to Nuremberg where he started a business of publishing mathematical and astronomical books, the first printing establishment specifically dedicated to scientific works.
According to David Lindberg in The Beginnings of Western Science,
“Reunion of the Roman Catholic and Greek Orthodox churches after centuries of schism brought Bessarion to Vienna in the 1460s, where he became the friend and patron of two young professors of astronomy: Georg Peurbach (1423-61) and Peurbach’s student Johannes Regiomontanus (1436-76). Motivated by the fall of Constantinople to the Turks in 1453, Bessarion campaigned to save as much of the Greek intellectual legacy as possible. One result was a Latin Epitome of the Almagest, produced by Peurbach and Regiomontanus – essentially a commentary that improved on all previous commentaries on Ptolemy’s Almagest and ‘provided Copernicus with a crucial conceptual stepping stone to the emergence of the heliocentric theory.’”
Nicolaus Copernicus (1473-1543) was born into a merchant family in the town of Toruń on the Vistula River in north-central Poland, south of the Baltic seaport of Gdańsk (Danzig) which was then the country’s largest city. He was educated at the university in Cracow or Kraków, which was for a long time the capital of the Kingdom of Poland, where he studied Latin, mathematics, astronomy, geography and philosophy. He spoke Polish and German fluently in addition to Greek and Italian, but the language of learning in Europe was Latin. Copernicus continued his education in Renaissance Italy between 1496 and 1503, studying Canon Law at the University of Bologna as well as medicine and astronomy at Padua, and finally took a degree at the University of Ferrara before returning to his native land.
Before 1514 he had written a small manuscript usually called the Commentariolus (“Little Commentary”), outlining some of his revolutionary heliocentric ideas, but this text was hand written and exclusively distributed to a few personal friends. Had it not been for his Austrian pupil, the astronomer Georg Joachim von Lauchen or Rheticus (1514-1574) from the University of Wittenberg, Copernicus’s masterpiece might never have been published.
Copernicus did carry out occasional planetary observations, but his system initially had little quantitative advantage over the Ptolemaic one. He preferred it because of its beauty and harmony. His model showed that the swiftest planet, Mercury, took up the orbit closest to the Sun whereas the slowest naked-eye planet, Saturn, fell farthest away. According to legend, Copernicus received a copy of his printed work De revolutionibus orbium coelestium (On the Revolutions of the Heavenly Spheres) on his own deathbed in 1543. His book was considered an important astronomical text even by many of those who did not believe in heliocentrism.
To Copernicus, his model was better than that of Ptolemy because it was more elegant. As James Evans notes, his theory contained a mixture of radical innovation and traditional astronomy:
“To launch the Earth into orbit was a bold move. The Sun-centered theory does have great explanatory advantages. And it does turn the whole solar system into a unified whole, as Copernicus himself stressed. But in the technical details of his planetary theory, Copernicus remained a part of the Ptolemaic tradition. Nearly every detail of his model has a corresponding element in Ptolemy’s model. It was for this reason that Kepler was later to say that Copernicus would have done better if he had interpreted nature, rather than Ptolemy.”
The ancient Greek astronomer Aristarchus of Samos was the first known person to maintain that the Earth revolves around the Sun. His ideas are mentioned in several preserved works such as Archimedes’s Sand Reckoner, which Copernicus was probably familiar with, and in the writings of the influential Greek historian Plutarch (ca. AD 46-120). As author David C. Lindberg states, “It is usually assumed that Aristarchus also gave the other planets sun-centered orbits, although the historical evidence does not address this point. In all likelihood, Aristarchus’s idea was a development of Pythagorean cosmology, which had already removed the earth from the center of the universe and put it in motion around the ‘central fire.’”
The heliocentric theory of Aristarchus from the third century BC was overwhelmingly rejected in Antiquity because it seemingly violated common sense, everyday observations and Aristotelian physics. If the Earth orbits the Sun, why can birds fly equally well in all directions? Another problem was that of stellar parallax, a relative change in the position of stars over a six month period as the Earth orbits the Sun, which could not be observed. Aristarchus stated that the stars are extremely far away and that the parallax is consequently too small to be observed. This is exactly the same answer that Copernicus gave much later.
Seleucus of Seleucia, a Hellenistic astronomer from Mesopotamia in the second century BC, was one of very few ancient scholars who supported Aristarchus’s heliocentric theory. Another possible, but significantly more controversial claim for pre-modern support of heliocentrism is the mathematical astronomer Aryabhata in early medieval India.
According to writer Michael H. Hart,
“The enormous scientific advances made by Europeans between 1540 and 1700 – we might call them collectively ‘the Scientific Revolution’ – dwarf even the noteworthy advances made by the ancient Greeks. Indeed, they are greater than all the scientific advances made throughout the entire world in all prior ages combined. One might truly say that science, as an organized human activity, came into existence in this period. What had previously been a sporadic activity, one occasionally indulged in by isolated persons, became an important, continuing project of a small but important community.”
This begs the question: What triggered the Scientific Revolution? Europe of the Late Middle Ages was increasingly prosperous. The rediscovery of the writings of the ancient Greeks and the introduction of the printing press were necessary conditions for the developments that took place, but not sufficient ones. Why were there more people engaged in mathematics and science during this age than before? Michael H. Hart speculates whether the introduction of the heliocentric hypothesis was responsible for this seemingly abrupt change in mentality.
While Copernicus did remove the Earth from the central place it had previously enjoyed in the cosmos it is important to realize that this did not automatically imply a lesser status since in the Greek worldview, the heavens were eternal and perfect whereas the Earth was the region of corruption and imperfection. As writer Rémi Brague notes,
“The Copernican hypothesis, far from being considered a wound, was felt as a flattering promotion: instead of crouching in a dungeon, man was henceforth the inhabitant of a neighborhood as chic as the sun’s.”
Nevertheless, there is no doubt that the heliocentric theory triggered a mental revolution, first in Europe and eventually throughout the world. It was not just another mathematical construct; it radically altered man’s place in the universe and – most importantly – his relations to God. This is why it triggered so much resistance of a non-scientific nature. In ancient times, many peoples had assumed that the Earth was the center of creation, and often that their particular city, country or nation was also the center of the Earth itself. The heliocentric theory removed the cradle of mankind from the center of the universe. In the centuries that followed, Western astronomers proved that the Sun was just one of countless stars and that the Milky Way is just one among countless billions of galaxies in the known universe. The most radical and controversial cosmologists in the twenty-first century even claim that our entire observable universe is just one among many different universes. Our tiny planet is thus far more insignificant in the cosmos than a grain of sand is to us. Copernicus himself would no doubt have been shocked by this realization, but he started the process which led to it.
The so-called Copernican Revolution was indeed a revolution, but it was a revolution that took generations to mature. Long after Nicolaus Copernicus had died there were still leading European astronomers who questioned his ideas, sometimes on a scientific basis.
The nobleman Tyco Brahe was born Tyge Brahe (1546-1601) in Scania (Skåne) in southern Sweden, then a part of the Kingdom of Denmark. He attended the universities of Copenhagen and Leipzig and traveled through the German-speaking lands, studying further at the universities of Wittenberg, Rostock and Basel. He lost his nose in a duel and wore an artificial nose made from silver and gold. With financial aid from the King of Denmark he set up Europe’s finest observatory called Uraniborg on the little island of Hven near Copenhagen, equipped with exceptionally large instruments plus an alchemical laboratory. In 1572 Brahe recorded the first modern European observation of a supernova, which undermined the Greek concept of a fixed sphere of unchanging stars and the Aristotelian division between the corrupt and ever changing sublunary world and the perfect and immutable heavens. He was also able to show that a comet he spotted in 1577 was further away than the planet Venus.
Tycho Brahe was influenced by some technical elements of the Copernican theory but developed an alternative geo-heliocentric system in which the planets all went around the Sun while the Sun moved around a stationary Earth. He opposed Copernicus for several reasons, one of them being his attachment to Aristotelian physics, another being the lack of observable stellar parallaxes. He changed observational practice profoundly. Whereas earlier astronomers had been content to observe the positions of the planets and the Moon only at important points of their orbits, Tycho and his assistants observed these bodies throughout their orbits. As a result, he detected a number of orbital anomalies that had never been noticed before.
“With his mural quadrant and other naked-eye instruments, Tycho recorded the positions of hundreds of stars and followed the motions of planets over decades. His mass of data was invaluable for later astronomers. Tycho’s measurements were the most accurate ever made until telescopes came on the scene.” As science historian John North states in his book Cosmos,
“Much of his early work is reliable to three or four minutes of arc, and his later accuracy is often better than a minute of arc for star positions, and hardly much less for those of the planets. This was better, by a factor of five or even ten, than the level of accuracy of the best Eastern astronomers, even than that of Ulugh Beg’s observatory in Samarqand.”
Ulugh Beg (ca. 1394-1449) was the grandson of the brutal and influential Islamic conqueror Timur, often known as Tamerlane (1336-1405). His father had captured the city of Samarkand in Central Asia where Ulugh Beg proceeded to build an observatory. With improved instruments and careful observations, he made a new star catalog with unprecedented accuracy for its time and even corrected some errors in Ptolemy’s calculations.
As Holy Roman Emperor, Rudolf II arguably contributed to the religious tensions that culminated in the damaging Thirty Years’ War (1618-48), but during his reign (1576-1612) Prague was a cultural center of East Central Europe, with a flowering of art and architecture. According to authors Robert Bideleux and Ian Jeffries,
“Prague became one of the musical capitals of Europe, thanks to Rudolf’s patronage of orchestras, choirs and polyphonic music. Last but not least, he built up a major art collection, including works by Leonardo da Vinci, Raphael, Tintoretto, Titian, Brueghel, Dürer, Holbein and Cranach. Intellectually, Rudolf’s Prague was home to the Czech founder of meridian astronomy, Tadeas Hajek of Hajek, the German mathematician and astronomer Johannes Kepler and the Danish astronomer Tycho Brahe, while in medicine Jan Jessenius pioneered dissection at Prague University.”
Tycho Brahe’s patron died and the new king was less positive. In 1597 he left Denmark and in 1600 went to Prague to the post as Imperial Astronomer at the court of Rudolf II. The great German astronomer and mathematician Johannes Kepler (1571-1630) joined him there from Graz in Austria, where anti-Protestant policies made his life difficult.
Kepler was a deeply religious Christian man who made numerous references to God in his works. At the University of Tübingen he had studied mathematics, Greek and Hebrew. Teaching was in Latin. He appears to have accepted quite early that the Copernican system was true, but through his work he was to discredit some ideas that Copernicus himself had maintained from the ancient Greeks, above all the concept that the planets move in perfect circles. Although Tycho had a large mass of observational material available he had not developed completed theories and was not forthcoming with his data at first. When Kepler arrived to work with him, Brahe’s principal assistant was observing Mars. Of the naked-eye planets, only Mercury and Mars have eccentricities large enough to make the departures of their orbits from perfect circles apparent from naked-eye observations, but Mercury is close to the Sun and difficult to observe. In essence, this means that Kepler’s discovery of the elliptical nature of planetary orbits could only have been made through a study of Mars.
After Tycho died in 1601, Kepler succeeded him as Imperial Mathematician. The process of calculating the orbit of Mars was immensely laborious but was completed around 1605. Meanwhile, Kepler did very valuable work on optical theory. His discoveries were finally published in 1609 in Astronomia nova (New astronomy), which was a difficult book to read as Kepler had not done a very good job of highlighting his most important results. Kepler’s first planetary law stated that the planets move in elliptical paths with the Sun at one focus, not in perfect circles as had been assumed by the ancient Greeks since Eudoxus of Cnidus.
Kepler’s second law stated that a line joining a planet to the Sun sweeps out equal areas in equal intervals of time as the planet describes its orbit, which implies that the planets move most rapidly when they are closest to the Sun. Kepler’s third law, which was published separately in 1619 a decade after the first two, stated that the square of the time of an orbit equals the cube of the mean distance from the Sun. At the same time, Galileo Galilei introduced the telescope to astronomy and discovered the largest moons of Jupiter. Kepler wrote an enthusiastic reply to Galileo’s work the Sidereal Messenger, published in 1610.
The discovery of a new astronomy resulted in the complete destruction of the old physics. The leaders of this development were Galileo Galilei, René Descartes and Isaac Newton.
According to James Evans,
“The area law, which is equivalent to the principle of conservation of angular momentum, provided the crucial clue that the force exerted on a planet by the Sun is directed radially inward toward the body of the Sun itself, and not tangentially around the orbit, as Kepler and his contemporaries had supposed. The elliptical shape of the orbit and, even more directly, the harmonic law provided the clues that the attractive force exerted by the Sun on a planet varies as the inverse square of the distance between them. For the ancient Greeks, planetary astronomy had been a branch of mathematics. Kepler’s constant goal was to provide a physical basis for astronomy. In his Mathematical Principles of Natural Philosophy of 1687, Isaac Newton showed how to deduce Kepler’s laws of planetary motion from his own laws of motion and the law of universal gravitation: it was Newton who realized Kepler’s dream of making planetary astronomy into a branch of physics.”
Kepler’s laws aided European astronomers in other ways, too. As author John Gribbin writes in his book The Scientists,
“In 1671 the French astronomer Jean Richer (1630-1696) travelled to Cayenne, in French Guiana, where he made observations of the position of Mars against the background of ‘fixed’ stars at the same time that his colleague in Paris, the Italian-born Giovanni Cassini (1625-1712), made similar observations. This made it possible to work out the distance to Mars, and, by combining this with Kepler’s laws of planetary motion, to calculate the distance from the Earth (or any other planet in the Solar System) to the Sun. The figure Cassini came up with for the Sun-Earth distance, 140 million km, was only 7 percent less than the accepted modern value (149.6 million km), and gave the first accurate indication of the scale of the Solar System. Similar studies of Venus during the transits of 1761 and 1769 (predicted by Halley) led to an improved estimate of the Sun-Earth distance (known as the Astronomical Unit, or AU) of 153 million km, close enough to the modern value for us to leave the later improvements in the measurements as fine tuning, and accept that by the end of the eighteenth century astronomers had a very good idea of the scale of the Solar System.”
Giovanni Cassini, a professor of astronomy at the University of Bologna, Italy, was invited by King Louis XIV of France to join the recently formed Académie Royale des Sciences and assumed the directorship of the Paris Observatory after it was completed in 1671. In the 1670s and 80s he discovered four of Saturn’s moons: Iapetus, Rhea, Tethys and Dione. He was able to measure Jupiter’s and Mars’s rotational periods and determined the parallax of Mars, which allowed the first realistic calculation of the distance to Mars and the Earth-Sun distance. He went on to establish a Cassini dynasty which dominated the Paris Observatory until the French Revolution. Giovanni Cassini is generally credited, perhaps along with the Englishman Robert Hooke, with the discovery of the Great Red Spot on Jupiter around 1665.
The Dutch astronomer Christiaan Huygens argued that if Sirius is as bright as the Sun it must be 27,664 times further away. By 1685, Newton used a new technique devised by the prominent Scottish astronomer and mathematician James Gregory (1638-1675) to show that the stars must lie at much greater distances from the Sun than had previously been supposed.
James Gregory was born near the city of Aberdeen in Scotland and studied there and well as at the University of Padua in Italy. He returned to London in 1668 and was soon appointed to the University of St. Andrews in Scotland and from 1674 to the University of Edinburgh as a professor of mathematics. As a mathematician he contributed to the development of calculus. In Optica Promota (“The Advance of Optics”) from 1663, Gregory introduced photometric methods to estimate (but not directly measure) distances to the stars and a description of a practical reflecting telescope known as the Gregorian telescope. He also pointed out the possible use of transits of Venus and Mercury to determine the distance to the Sun, something which was successfully done after his death.
Isaac Newton had to show that the stars were so far away that their gravitational attractions on one another were minimal. This was important to him as he wondered why the world does not collapse on itself, under gravity. We now know that the universe is expanding, but this was proven by Western astronomers only in the twentieth century, long after Newton had died.
Some cosmologists have suggested the possibility that the universe may in fact collapse at some point in the future due to gravity and end in a singularity. Although this Big Crunch, the opposite of the Big Bang, is one possible, hypothetical fate of the universe it is not the only conceivable future and according to observational evidence not necessarily the most likely one. Indeed, some observations from the late 1990s and afterward could indicate that the expansion of the universe is not being slowed down by gravity but is rather accelerating.
The English astronomer James Bradley (1693-1762) discovered stellar aberration while looking for stellar parallax. Since the diameter of the Earth’s orbit is roughly 300 million kilometers, nearby stars should appear to move compared to more distant ones over a six-month period as the Earth orbits the Sun. They do, but because the stars are extremely far away from us this effect is difficult to measure. While studying the star Gamma Draconis, Bradley in 1728 succeeded in detecting a slight annual variation in the apparent positions of stars, but in the opposite direction from what was expected. This is caused by the aberration of light, a result of the finite speed of light and the forward movement of the Earth in its orbit around the Sun. This finding provided the first direct evidence for the Copernican theory.
The fact that the speed of light is finite had been known in Europe since the work of the astronomer Ole Rømer from Denmark in 1676. Combined with increasingly accurate knowledge of the Earth-Sun distance, James Bradley could correctly calculate that it takes sunlight more than eight minutes to travel from the Sun to observers here on the Earth.
The German scholar Friedrich Wilhelm Bessel (1784-1846) was born in Minden and despite his limited education became one of the leading astronomers of his generation, a rigorous observer as well as a capable mathematician. He was a contemporary of the mathematician Carl Friedrich Gauss and was brought into the field of astronomy in 1804 when he contacted the great German astronomer Wilhelm Olbers concerning a paper he had written on Halley’s Comet. In 1836 he published a theory which stated that comets consist of volatile matter.
Bessel’s contributions covered most of contemporary astronomy, especially precision measurements. Aided by better instruments as well as his own personal skills he won the race to be the first to measure stellar parallax and by implication the distance to a star other than the Sun. He spent years observing and accurately pinpointing the positions of thousands of stars, systematizing the observations of James Bradley. Bessel’s efforts culminated in 1838 when he calculated the distance to the star 61 Cygni as more than 10 light-years. This has later been modified to about 11.4 light-years. He chose a star with unusually large proper motion, correctly deducing that it was probably close to us by astronomical standards.
The Baltic German astronomer Friedrich Struve (1793-1864) and the Scottish astronomer Thomas Henderson (1798-1844), too, measured stellar parallax at almost the same time, but Bessel’s measurements were most convincing. Henderson measured the distance to Alpha Centauri, the nearest stellar system to our own at about 4.4 light-years away from our Sun.
During his many years at the Königsberg Observatory in Prussia, Bessel’s students included the German-born astronomer Friedrich Wilhelm August Argelander (1799-1875), who had a German mother and a Finnish father. Argelander established the study of variable stars as an independent branch of astronomy and was appointed director of the Turku Observatory in southwestern Finland in 1823 and of the Helsinki Observatory in 1832. He then moved to Bonn, Germany and published an extensive star catalog there in the 1850s.
The Scottish astronomer David Gill (1843-1914) was born in Aberdeen, Scotland and educated at the University of Aberdeen, but he spent many years of his career in South Africa where he also made geodetic surveys. He was trained and worked as a watchmaker, and his interest in timekeeping led to an interest in astronomy. Gill used the parallax of Mars to re-determine the distance to the Sun with such precision that his value was used for almanacs until 1968. He carried out observations of stellar parallax to measure distances to other stars.
The Hipparcos satellite of the European Space Agency (ESA) in the early 1990s carried out tens of thousands of accurate measurements of star positions and parallaxes. ESA’s Gaia Mission will create even more accurate three-dimensional maps over many stars after 2012.